Earth is heating up: “Global surface temperature was 1.09°C higher in 2011– 2020 than 1850–1900,” according to the Intergovernmental Panel on Climate Change (IPCC)’s 6th Assessment Report.[3] Yet the mercury is not rising uniformly around the world – the Arctic is warming faster than are the lower latitudes, and temperatures over land are higher than over the ocean. Local temperatures everywhere are also affected by the type of land cover, with paved areas being hotter than vegetated land.
In Phoenix, Arizona, the temperature topped 110°F during the summer of 2020 – not just once, but for 53 days.[4] In the same year, Los Angeles experienced its hottest day ever: 122°F. Paris’ hottest day record was broken in 2019, and Buenos Aires’ second and third hottest days ever were in January 2022 (its hottest was in 1957). While climate change is to blame for the world’s increasing frequency of heatwaves, cities additionally experience the “urban heat island” (UHI) effect, which can add as much as 20°F compared to their rural surroundings.[5]
To deal with heatwaves, the city of Phoenix established a new team to manage the public health effects of heat and find ways to reduce it. Part of the plan is doubling the city tree canopy to 25% coverage. Urban heat islands are created by a low ratio of vegetated surfaces relative to concrete, asphalt, and other unvegetated and impervious surfaces, which absorb sunlight as heat and radiate it back out. By contrast, plants use the sun’s energy to transpire water into the atmosphere, a process that cools rather than heats the surrounding air.
Many cities are planting trees to dampen scorching summer heat as public awareness of the relationship between vegetation and the UHI grows. Expanding public green space and tree canopy is a practical way for localities to adapt to the juggernaut of a changing climate because increased vegetation cover not only lowers local temperatures, but can also reduce the severity of flooding and drought by improving rainwater infiltration.
Just as increasing tree cover reduces temperatures in cities, reforestation has a cooling effect at regional and even global scales. The studies profiled below explore a variety of aspects of the relationship between vegetation types, especially forests, and temperature. All produced in the past ten years, these studies show that reforestation (establishing forest on previously forested land) and afforestation (foresting land that was not previously in forest) can lower temperatures, and that deforestation, conversely, has raised temperatures across vast areas.
For example, historical conversion of natural forests in the midlatitudes to cropland and pasture has more than tripled the occurrence of hot-dry summers in those areas [Findell 2017]. Clearing forests heats the land not only by releasing CO2, which contributes to the greenhouse effect, but also changes the albedo (reflectivity), surface roughness, and evapotranspiration (ET) rates of land surfaces. Land-cover changes affect the temperature through biophysical exchanges of water and energy between the land and the atmosphere.
By 1920, the biophysical effects of deforestation were the main cause of increasing temperatures in deforested parts of North America and Eurasia [LeJeune 2018]. Over the 20th Century, greenhouse gases started to play a bigger role in regional heating. Today, the combined effects of the biophysical changes and greenhouse gas emissions wrought by deforestation account for an estimated half of the temperature rise since pre-industrial times in deforested areas of the midlatitudes [LeJeune 2018].
Interestingly, the presence of forests affects surface temperatures differently depending on latitude, although globally, vegetation gain has a net cooling effect [Piao 2020]. In boreal regions, forestation is associated with a warming effect due to a lower albedo of forests compared to non-forest land. By contrast, deforestation warms the mid-latitudes and tropics – in spite of the lower surface albedo of forested land. The cooling effect of ET drives temperature regulation in the tropics, while both ET and cloud formation from forests cool the mid-latitudes.
The Jambi province of tropical Sumatra, Indonesia, warmed an average of 1.05°C between 2000 and 2015, a period that coincided with rapid deforestation [Sabajo 2017]. The forests that remained in Jambi during this period also warmed slightly, but much less so than the region as a whole. The authors suggest that the smaller temperature rise inside forests reflects global warming, while the greater regional warming is due to the combined effect of global warming and the biophysical effects of local deforestation.
Vegetation cover both affects and is affected by climate change. Piao et al. [2019] documented an increase in global greening since 1980, which they attribute in part to growth-inducing impacts of climate change. Warmer temperatures and the fertilization effects of higher CO2 concentration, but also nitrogen deposition and direct afforestation efforts, all have increased vegetation cover, though not evenly across the planet, and not necessarily where it would have the greatest cooling effect. For example, warmer temperatures increase vegetation in the northern latitudes by lengthening the growing season, but reduce vegetation in the tropics where historical temperatures are already optimal for plant growth.
In addition to modulating temperatures, forests also influence precipitation. Through ET – the very same mechanism responsible for cooling the land – forests recycle water back into the atmosphere. Globally, at least 40% of the rain that falls on land comes from evapotranspiration on land, while as much as 70% of rain originating over the Amazon is ET-fed [Ellison 2017]. In Europe, converting a limited proportion (not expected to impinge on food security) of agricultural lands would increase rainfall on the continent by an estimated 7.6%, an important augmentation given the growing frequency of drought there [Baker 2021].
The role that forests and other natural vegetation types play in mitigating global warming and softening the blow of climate extremes has not always been universally clear, but this is changing. The studies highlighted below, which illustrate the heat- and drought-limiting capacity of forests, are among a growing body of work pointing to the importance–and power–of natural climate solutions.
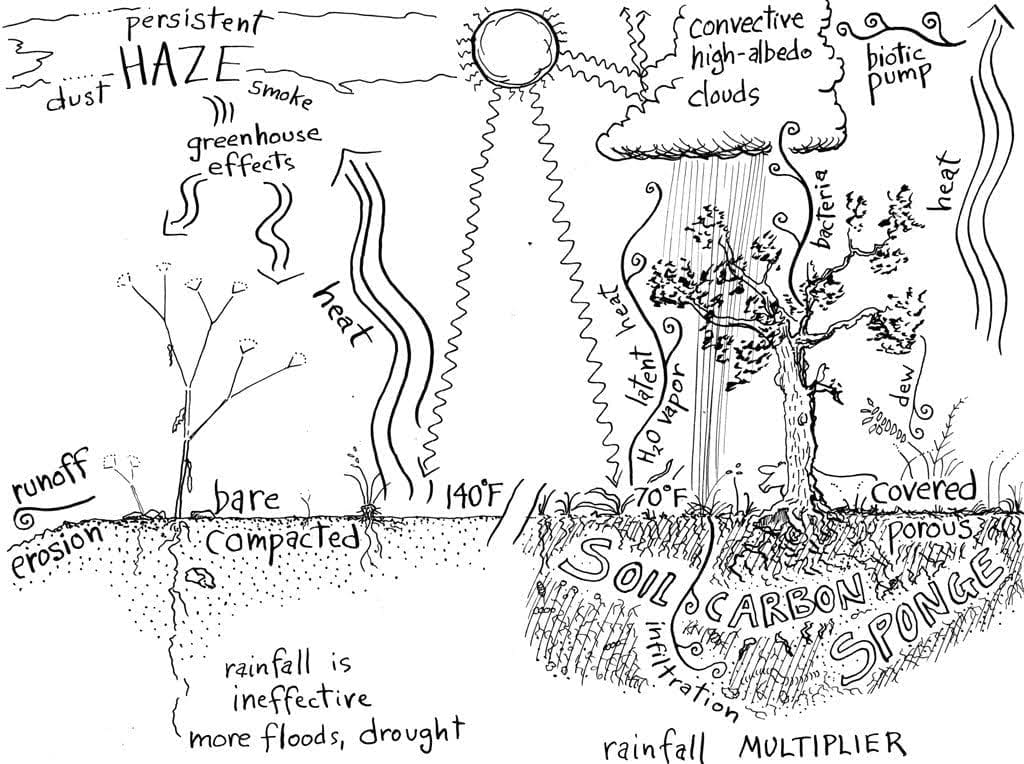
Summaries of articles showing the cooling effect of vegetation
Cloud cooling effects of afforestation and reforestation at midlatitudes, Cerasoli, Jin & Porporato 2021
Reforestation and afforestation (R&A) are well-established climate mitigation strategies in the wet tropics due to high carbon sequestration rates of forests/trees. However, at high latitudes (boreal regions), the low albedo of trees–compared to snow and other lighter land surfaces–leads to the absorption of energy, thus creating a warming effect that has a greater impact on temperature than the carbon capture accomplished by the limited vegetation productivity in boreal regions. This study explores the balance between albedo and carbon sequestration of forests at mid-latitudes, which has been less clear.
The authors found that forested areas have greater cloud cover than other types of land cover at midlatitudes, resulting in a higher albedo at the top of the atmosphere–where the clouds are–and leading to greater cooling. Specifically, they found “an association of forested lands with increased cloudiness… As a result, forests reflect extra solar radiation and thus reduce the radiative impacts of the lower surface albedo. This in turn implies a cooling effect of R&A at midlatitudes” [Cerasoli 2021: 1-2]. The increase in cloudiness is due to earlier afternoon cloud formation over forests compared to other vegetation types in wet regions.
Our results provide substantial evidence of remarkable benefits of R&A [reforestation and afforestation] around the 30° to 45° latitudinal range, due to the combined benefits of biomass gain and promotion of cloud formation over forests [Cerasoli 2021: 4].
The duality of reforestation impacts on surface and air temperature, Novick & Katul 2020
While reforestation has been widely heralded as a means of sequestering carbon into the soil, there is growing evidence that it also serves to directly cool the land surface. But forests’ impacts on air temperature (measured over forests rather than within them) have been difficult to assess because of the confounding impacts of forest canopies on wind and temperature profiles near the surface. This study was implemented to create a new method for assessing to what degree forests also cool the air.
Most studies measure surface temperature, which “represents the aggregated temperature of solid canopy and soil elements,” and is measured at a midway point between the ground and the top of the canopy. Air temperature, on the other hand, is measured above the vegetation canopy (whether grasslands or forest). The study site, located in the Piedmont region near Durham North Carolina, consists of an old-field grassland, a pine forest, and an unevenly aged oak hickory forest, all within close proximity to each other. The study assessed temperatures at various heights in and above the grasslands and the two forests.
The authors found that surface temperatures are much lower in forests than in grassland; this difference often exceeds 5°C at midday during the growing season. Furthermore, the air is cooler over forests than over grasslands, though to a lesser degree than the surface temperature difference. The annual average air temperature difference of forests compared to grassland is 0.5°C to 1°C, while the difference reaches 2°C to 3°C during daytime growing season periods.
“Making the connection between land cover, surface temperature, and air temperature is becoming necessary for obtaining a complete picture of the climate mitigation and adaptation potential of managed land cover changes, including reforestation,” the authors conclude. “This energy balance perspective on the climate mitigation and adaptation potential of reforestation is especially relevant right now” given a recent global surge of interest in reforestation to sequester carbon [Novik & Katul 2020: 13].
Characteristics, drivers and feedbacks of global greening, Piao et al. 2019
The amount of Earth’s green cover (measured as Leaf Area Index[6]) has increased globally since 1980, especially in northern latitudes, where growing seasons have lengthened. This is due mainly to increasing CO2 concentration, but also to warmer temperatures and changing precipitation patterns, nitrogen deposition, and land-use change (such as afforestation in China). Higher ambient CO2 can stimulate photosynthesis and reduce water loss, but the extent of the CO2 fertilization depends on the availability of other key nutrients (nitrogen and phosphorus) and water. Warmer temperatures due to climate change have increased greening in northern latitudes by extending the growing season, but diminished greening in the tropics, where temperatures were already optimal.
Greater global green cover has observable feedbacks on climate and the carbon cycle. In addition to offsetting 28% of anthropogenic emissions since 1980, vegetation affects hydrological cycles and air-surface temperatures. Since the 1980s, increased global evapotranspiration (ET) is mainly attributable to increased global greening. Higher transpiration rates from vegetation can reduce or enhance groundwater storage locally, depending on how the atmospheric moisture generated through evapotranspiration is recycled into rain and where that rain falls. In the world’s great rainforests, vegetation preserves groundwater.
The enhanced precipitation over transpiring regions is particularly evident in moist forests like the Amazon or Congo, which are ‘closed’ atmospheric systems where 80% of the rainfall originates from upwind ET. Such an efficient atmospheric water recycling mitigates water loss from the soil, sustains inland vegetation and maintains mesic[7] and humid ecosystems [Piao 2019: 9].
Vegetation affects land-surface temperature by way of ET (cooling effect) and albedo (warming or cooling effect, depending on how dark or light the surface is). While the relative strength of ET versus albedo varies by latitude, the net global effect of increasing vegetation cover is one of cooling the land surface.
Historical deforestation locally increased the intensity of hot days in northern mid-latitudes, Lejeune 2018
Deforestation has contributed to warming in the northern mid-latitudes of North America and Eurasia not only through a large contribution to global CO2 emissions, but also through biogeophysical effects. The latter refers to land-surface effects such as albedo and evapotranspiration, which vary according to the type of land cover. This study uses models to demonstrate that deforestation in the northern mid-latitudes has increased the intensity of hot days by about a third since pre-industrial times. Factoring in deforestation’s contribution to greenhouse gas emissions further increases deforestation’s heating effect.
Our best estimate suggests that the present-day contribution of deforestation to the TXx [yearly maximum temperature, or “hot days”] increase over this region still equals at least 50% once the warming entailed by the LCC [land cover change]-induced carbon emissions is considered [LeJeune 2018: 4].
“Extensive deforestation took place early in the industrial period over the northern mid-latitudes,” and then slowed down in the 20th Century [LeJeune 2018: 4]. By 1920, modeled increases of temperature “through biogeophysical effects had already reached 0.3°C (~75% of their present-day values) over the most deforested areas of North America and Eurasia. On average before 1920, local deforestation was responsible for most of the TXx [yearly maximum temperature] warming over these regions” [LeJeune 2018: 4]. Warming caused by greenhouse gases became more important during the 20th Century, “leading to a total warming of 1.3°C over North America and 1°C over Eurasia by the present-day” [LeJeune 2018: 4].
Expansion of oil palm and other cash crops causes an increase of the land surface temperature in the Jambi province in Indonesia, Sabajo 2017
Turning lemons into lemonade, Sabajo et al. have used the great expansion of oil palm plantations and other crops in Indonesia to examine how such land-use change affects land surface temperature (LST). The authors observed a warming trend in the Jambi province of Sumatra of 1.05℃ and 1.56℃ in the morning and afternoon, respectively, between 2000 and 2015. The average morning (10:30 am) temperature increased by 0.07℃ per year; the midday afternoon (1:30 pm) temperature increased by 0.13℃ per year.
During roughly the same period (2000-2010), forest area decreased in Jambi by 17%, while oil palm and rubber plantations greatly expanded. Given that LST within the province’s remaining forests increased only .04C per year at 10:30 am, which the authors attribute mainly to global warming, they concluded that the overall higher province-wide daytime temperature increase was caused by the observed land cover change.
The team also compared temperatures between different land uses: forest, oil palm and rubber plantations, urban areas, and bare land. Despite having a higher albedo (reflectivity) than the forest areas, all converted (non-forest) lands were nonetheless warmer than forests, “suggesting that the albedo was not the dominant variable explaining the LST” [Sabajo 2017: 4629]. Evapotranspiration (ET) played a greater role. Non-vegetated surfaces (urban and bare) were the warmest.
The authors conclude: “The warming effect after forest conversion results from the reduced evaporative cooling, which was identified as the main determinant of regulating the surface temperature” [Sabajo 2017: 4631].
The impact of anthropogenic land use and land cover change on regional climate extremes, Findell et al. 2017
This paper analyzes how land use and land cover change (LULCC) affects temperature and humidity. The authors examined the differential effects of forest versus deforested land on temperature and humidity by comparing different land-cover models. One model simulated the total potential vegetation (“PotVeg”) that would cover Earth in the absence of human interference, while the other was based on historical data of land use changes that occurred over a recent five-decade period (“AllHist”).
They found that deforested lands in mid-latitudes (North America, Eurasia) in the AllHist model are warmer and drier compared to the same lands covered in forest in the PotVeg model. Specifically, “conversion of mid-latitude natural forests to cropland and pastures is accompanied by an increase in the occurrence of hot-dry summers from once-in-a-decade to every 2–3 years” [Findell 2017: 1]. “Based on these simulations, the conversion of forests to cropland is coincident with much of the upper central US and central Europe experiencing extreme hot, dry summers” [Findell 2017: 6].
Local temperature response to land cover and management change driven by non-radiative processes, Bright et al. 2017
Local temperatures are affected not only by global climatic factors, but also by radiative (albedo) and non-radiative (evapotranspiration and convection) mechanisms related to local vegetation cover. Through evapotranspiration, solar energy is converted to latent heat and released from the planet’s surface, while convection refers to the turbulent mixing of air that dissipates sensible heat. The authors state that while albedo (reflectivity of land surface, which is often lower on forested land) is increasingly accounted for alongside greenhouse gases in climate models, the non-radiative mechanisms are not. However, the evapotranspiration and convection facilitated by vegetation have an important cooling effect and should therefore be included in models to avoid the risk of “promoting land sector policies that may be counter to the aims of mitigation or adaptation” [Bright 2017: 296].
The authors demonstrate that “non-radiative mechanisms dominate the local response in most regions for eight of nine common LCMC perturbations” [Bright 2017: 296]. Land cover and land management changes (LCMC) considered in the study include converting cropland or grassland to deciduous or evergreen forests. The authors found that gains in forest cover increased annual cooling in all but the northernmost latitudes, where the lower albedo of forests compared to grasslands had a warming effect that was stronger than the cooling effect of non-radiative mechanisms. In many regions, including much of Europe, the US, and the tropics, non-radiative cooling dominated albedo effects. “Over annual timescales, forest cover gains result in net cooling for many of the densely populated regions of the planet” [Bright 2017: 298].
Bright et al. conclude that “benchmarking the locally driven LCMC effect to that driven by global forcers (such as CO2) can provide an additional perspective by which to support the valuation of vegetated ecosystems and the local climate regulation services that they provide” [Bright 2017: 301].
Trees, forests and water: Cool insights for a hot world, Ellison et al. 2017
This article (also highlighted in Compendium v2n1) reviews research on the benefits of tree cover in relation to water and energy cycles.
Forests help produce rain. Vegetation releases water vapor through transpiration, increasing atmospheric moisture that is then transported by wind. In fact, “over most of the tropics, air that passes over forests for ten days typically produces at least twice as much rain as air that passes over sparse vegetation” [Ellison 2017: 53]. Forests also release biological particles, such as spores, bacteria and pollen into the atmosphere. Water condenses around these particles, forming raindrops.
In addition to the atmospheric moisture produced by forests that is transported by prevailing winds to generate downwind rain, forests also help transport moisture from the coasts to the interior of continents. According to the biotic pump theory [Makarieva & Gorshkov 2007], evapotranspiration over coastal forests creates low pressure zones that draw in atmospheric moisture from the ocean. This oceanic moisture eventually comes down as rain over land. Deforestation of coastal forests thus reduces this influx of moisture to land, while deforestation anywhere can decrease the reliability of rainfall downwind.
Through shading and evapotranspiration, forests cool the Earth’s surface in tropical and temperate climates. Due to a lower albedo compared to other land cover types at high latitudes, boreal forests potentially contribute to local warming. However, forests also increase cloud cover and thus albedo, higher in the atmosphere. In the absence of vegetation, such as in cities, solar energy remains in the environment in the form of heat, rather than driving evapotranspiration.
Using the sun’s energy, individual trees can transpire hundreds of liters of water per day. This represents a cooling power equivalent to 70 kWh for every 100 L of water transpired (enough to power two average household central air-conditioning units per day) [Ellison 2017: 54].
High-elevation forests have a unique potential to intercept fog and cloud droplets, which boosts tree growth, evapotranspiration, groundwater infiltration, and ultimately contributes up to 75% of catchment runoff. Tree cover can improve water infiltration due to increased organic matter to hold water and the presence of tree roots, which loosen and shade the soil and channel water into the ground. In areas where infiltration rates are greater than transpiration rates, the presence of trees increases groundwater recharge.
All of the aforementioned mechanisms distribute water naturally, hence reducing floods.
Baker, Jessica C. A., 2021, Planting trees to combat drought, Nature Geoscience 14, https://doi.org/10.1038/s41561-021-00787-0.
Bright, Ryan M., et al., 2017, Local temperature response to land cover and management change driven by non-radiative processes, Nature Climate Change 7, http://doi.org/10.1038/NCLIMATE3250.
Cerasoli, Sara, Jun Yin & Amilcare Porporato, 2021, Cloud cooling effects of afforestation and reforestation at midlatitudes, PNAS 118(33), https://www.pnas.org/content/118/33/e2026241118.
Ellison, David, et al., 2017, Trees, forest and water: Cool insights for a hot world, Global Environmental Change 43, https://www.sciencedirect.com/science/article/pii/S0959378017300134?via%3Dihub.
Findell, Kirsten, et al., 2017, The impact of anthropogenic land use and land cover change on regional climate extremes, Nature Communications 8(989), https://www.nature.com/articles/s41467-017-01038-w.
LeJeune, Quentin, et al. 2018, Historical deforestation locally increased the intensity of hot days in northern mid-latitudes, Nature Climate Change 8(5), https://doi.org/10.1038/s41558-018-0131-z.
Makarieva, A. M. and V.G. Gorshkov, 2007, Biotic pump of atmospheric moisture as driver of the hydrological cycle on land, Hydrology and Earth Systems Sciences 11, https://hess.copernicus.org/articles/11/1013/2007/.
Piao, Shilong, et al., 2020, Characteristics, drivers and feedbacks of global greening, Nature Reviews Earth & Environment, https://www.nature.com/articles/s43017-019-0001-x.
Sabajo, Clifton R., et al., 2017, Expansion of oil palm and other cash crops causes an increase of the land surface temperature in the Jambi province in Indonesia, Biogeosciences 14, https://doi.org/10.5194/bg-14-4619-2017
[3] https://www.ipcc.ch/report/ar6/wg1/
[4] https://www.theguardian.com/us-news/2022/jan/27/phoenix-arizona-hottest-city-cooling-technologies
[5] https://nihhis.cpo.noaa.gov/Urban-Heat-Islands/Understand-Urban-Heat-Islands
[6] Leaf Area Index (LAI) is the quantity of leaf area per unit ground surface area; it’s a way to quantify the thickness of a vegetation canopy.
[7] Mesic refers to moderate moisture levels.